Solid-state batteries (SSBs) are at the center of the most significant technological leap in electric vehicle (EV) energy storage as of 2025, promising to overcome the limitations of todayโs lithium-ion batteries (LIBs) with breakthroughs in range, charging speed, and safety.
As of 2025, this is the current state of SSB science, global supply chain dynamics, and the environmental implications of sourcing critical materials like lithium and sulfur-a pivotal shift as the industry prioritizes Scope 2 and 3 emission reductions.
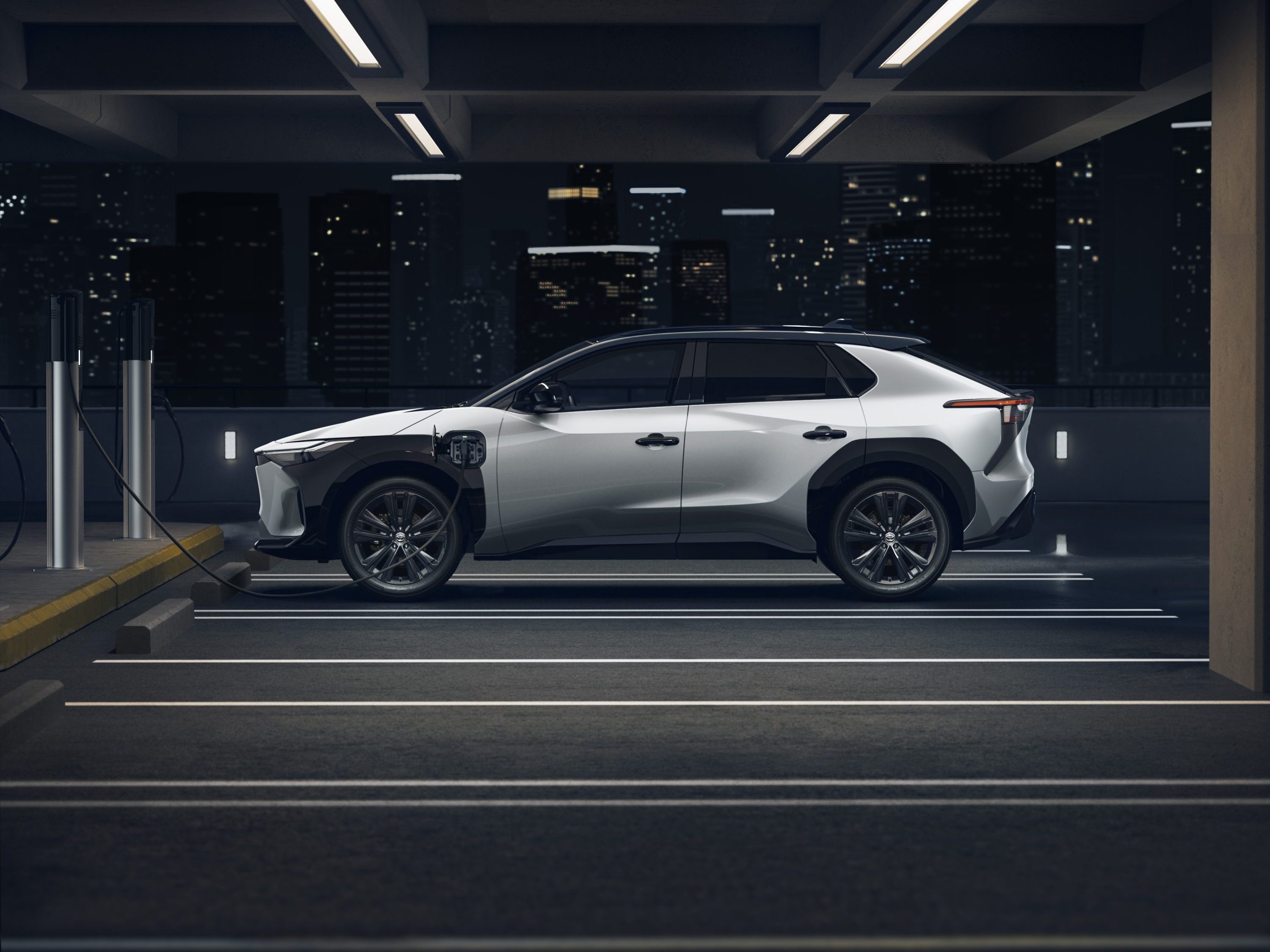
Unlike conventional LIBs, which use flammable liquid electrolytes, SSBs employ solid ceramic or polymer electrolytes, enabling much higher energy density, rapid charging, and greater thermal stability.
This is the current state of SSB science and technology, with a focus on Toyotaโs industry-leading efforts-such as its batteries promising up to 621 miles of range and charging in under 10 minutes-as well as the progress of other global automakers pursuing similar advancements.
Beyond performance, research examines environmental impact of SSBs, including how sourcing materials and manufacturing processes affect Scope 2 and Scope 3 emissions.
As automakers like Toyota, MG, and IM Motor move closer to commercializing SSBs, understanding their scientific foundations, sustainability implications, and the challenges of scaling up production is crucial for assessing the future of electric mobility.
These advancements are supported by breakthroughs in materials science, including atomic-layer-deposited electrolytes and crystalline organic compounds that enhance ionic conductivity while eliminating thermal runaway risks.
The technology is now transitioning from lab-scale prototypes to automotive validation, with companies like Stellantis, Mercedes-Benz, and Hyundai bringing scientific competition to Toyota’s own breakthrough processes.
Stellantis and Factorial Energy recently validated 77Ah SSB cells with 375 Wh/kg energy density and operation across extreme temperatures (-30ยฐC to 45ยฐC), targeting demonstration fleets by 2026.
Meanwhile, Hyundaiโs โDreamโ battery initiative aims to unveil its first SSB-powered prototype vehicle by late 2025, leveraging sulfide-based electrolytes for improved cost-effectiveness.
Despite these strides, challenges persist. Scalability remains hindered by dendrite formation at lithium-metal anodes and the moisture sensitivity of sulfide electrolytes, which demand costly dry-room manufacturing.
Production costs for SSBs are still 2โ3ร higher than LIBs, though innovations like gigacasting and solvent-free electrode processing aim to close this gap by 2030.
How Solid-State Batteries Work
Solid-state batteries (SSBs) and conventional lithium-ion batteries (LIBs) both store and release energy by moving lithium ions between the anode and cathode. The key difference lies in the electrolyte: SSBs use a solid electrolyte (often ceramic, polymer, or glass), while LIBs use a flammable liquid or gel electrolyte.
In an SSB, lithium ions move through a solid electrolyte during charging and discharging cycles. When charging, lithium ions travel from the cathode through the solid electrolyte and are stored in the anode, often made of lithium metal.
During discharge, the ions move back to the cathode, generating an electric current. The solid electrolyte acts as both the ionic conductor and a separator, reducing the risk of short circuits and eliminating the fire hazard associated with liquid electrolytes.
Comparison to Lithium-Ion Batteries in Cars
Feature | Solid-State Batteries (SSB) | Lithium-Ion Batteries (LIB) |
---|---|---|
Electrolyte | Solid (ceramic, polymer, glass) | Liquid or gel (flammable) |
Energy Density | Higher (350โ700 Wh/kg) | Lower (150โ300 Wh/kg) |
Safety | Much safer, no thermal runaway | Risk of fire/thermal runaway |
Charging Speed | Faster (12โ15 min to 80%) | Slower (30โ45 min to 80%) |
Operating Range | Wider temperature, higher voltage | More limited temperature, lower voltage |
Cycle Life | Potentially longer (100,000 miles) | 60,000 miles (typical) |
Cost (2025) | $800โ$1200 per kWh (high) | $100โ$150 per kWh (low) |
Commercial Status | Early stage, limited availability | Mature, mass-produced |
SSBs offer higher energy density, faster charging, greater safety, and broader operating conditions than LIBs.
SSBs are currently more expensive and harder to manufacture at scale, but costs are expected to fall as technology matures.
LIBs are widely used today due to established production and lower cost, but SSBs are seen as the next major leap for EVs.
The Science Behind Solid-State Battery Development
Toyotaโs SSB research focuses on sulfide-based solid electrolytes, which offer high ionic conductivity and compatibility with lithium-metal anodes. The company has achieved energy densities of approximately 1,000 Wh/L in prototypes, a 40% improvement over current LIBs.
By eliminating liquid electrolytes, Toyotaโs SSBs reduce thermal runaway risks, a critical safety advantage. Early road tests in modified EVs demonstrate ranges exceeding 745 km (463 miles) on a single charge, with charging times under 10 minutes.
“For both our liquid and our solid-state batteries, we are aiming to drastically change the situation where current batteries are too big, heavy and expensive. In terms of potential, we will aim to halve all of these factors.โ Keiji Kaita, president of the Toyota research and development center for carbon neutrality.
Toyota initially targeted SSB deployment by 2022 but faced delays due to manufacturing complexities. Recent prototypes suggest a revised timeline, with limited production expected by 2027โ2028 and mass adoption by 2030.
The company plans to integrate SSBs into luxury sedans and SUVs first, leveraging higher margins to offset initial costs.
Dendrite formation at the anode-electrolyte interface remains a key hurdle, as lithium filaments can short-circuit cells.
Toyota is exploring polymer coatings and hybrid electrolyte designs to mitigate this issue. Sulfide electrolytes are sensitive to moisture, requiring dry-room manufacturing-a costly infrastructure investment.
Competing Automakers in Solid-State Battery Development
Major automakers are investing heavily in SSBs to secure a foothold in the next-generation EV market. These are the key players:
Manufacturer | SSB Partner | Expected Launch | Targeted Vehicle Models |
---|---|---|---|
Toyota | In-house | 2027โ2028 | Lexus Electrified Sedan, bZ5X SUV |
Nissan | In-house | 2028โ2029 | Ariya successor, electric pickup |
Mercedes-Benz | Factorial Energy | 2026โ2027 | EQS Sedan, EQG SUV |
BMW | Solid Power | 2030+ | Neue Klasse Sedan, i7 successor |
Honda | SES AI Corp | 2028 | Prologue EV lineup |
Nissan leads in pilot production, with a dedicated SSB line operational since 2025. Its proprietary oxide-based electrolyte aims for 1,300 Wh/L energy density, targeting mid-market EVs.
Mercedes-Benz, collaborating with Factorial Energy, has integrated SSBs into an EQS prototype achieving 1,000 km (621 miles) of range.
BMW cites cost barriers, estimating SSB adoption no earlier than 2030.
Material Sourcing
SSBs require 35% more lithium than LIBs but significantly less graphite and cobalt.
Toyotaโs sulfide electrolytes use abundant sulfur, reducing reliance on conflict minerals like cobalt. By contrast, conventional NMC (nickel-manganese-cobalt) batteries depend on environmentally destructive mining practices, particularly in the Democratic Republic of Congo (cobalt) and Indonesia (nickel).
The carbon footprint of SSBs varies dramatically based on lithium sourcing:
Geothermal brine extraction (e.g., Germanyโs Upper Rhine Valley) emits 75% less COโ than Australian hard-rock mining.
Sedimentary clay mining, common in China, requires energy-intensive leaching, resulting in 2ร higher emissions than brine methods.
Toyota Tsusho, Toyotaโs trading arm, has prioritized partnerships with geothermal lithium startups since 2008 to secure low-carbon lithium. This aligns with Toyotaโs goal to reduce Scope 3 emissions by 30% by 2030, as outlined in its 2024 North American Environmental Sustainability Report.
Japanโs reliance on imported lithium (100% as of 2008) prompted Toyota to invest in diversified sources, including South American brine deposits and Australian spodumene.
The Inflation Reduction Actโs (IRA) emphasis on localized battery production has further driven Toyota to explore U.S.-based geothermal lithium projects, which could reduce transportation-related emissions by 18โ22%.
โI want to change the future of car-making through Toyotaโs skill. To achieve this, we need to evolve the monozukuri strengths that only Toyota possesses through the fusion of skills/technology and digital & innovative technologies, and to shorten lead times, to be agile and continually take on challenges.โ – Kazuaki Shingo, Chief Production Officer, Toyota.
Carbon Footprint, Scope 2 and 3 Emissions
Lifecycle analyses show SSBs can reduce battery carbon footprints by 24โ39% compared to LIBs, contingent on sustainable lithium sourcing.
For example, lithium extracted from geothermal brines (e.g., in Germanyโs Upper Rhine Valley) emits 75% less COโ than Australian hard-rock mining.
Toyotaโs partnership with geothermal lithium startups aligns with its goal to cut Scope 3 emissions by 30% by 2030.
SSBs exhibit a 24โ39% lower GWP than nickel-manganese-cobalt (NMC) lithium-ion batteries when using sustainably sourced lithium.
NMC-811 LIBs: ~280 kg COโeq/kWh (primarily from nickel sulfate and graphite).
SSBs with geothermal lithium: ~170 kg COโeq/kWh.
Toyotaโs collaboration with Idemitsu Kosan to produce lithium sulfide-a key SSB electrolyte-at a new $142 million plant in China aims to cut emissions by centralizing material processing. The plantโs coal-heavy energy mix in China risks offsetting gains, highlighting the need for renewable-powered facilities.
SSBsโ solid electrolytes complicate recycling versus LIBs. In comparison:
LIBs: Achieve 70% material recovery via pyrometallurgy, but cobalt and nickel recycling remains energy-intensive.
SSBs: Require hydrometallurgical methods to separate lithium and sulfur. Toyota targets 90% material reuse by 2035 through solvent-based recovery systems.
Dendrite-contaminated lithium anodes in spent SSBs pose safety risks, necessitating inert gas environments during dismantling-a process 30% costlier than LIB recycling.
Toyotaโs 2024 sustainability report highlights a 14% reduction in Scope 1/2 emissions since 2019, driven by renewable energy adoption in battery plants.
For Scope 3 (supply chain), the companyโs shift to sulfide electrolytes reduces reliance on cobalt suppliers linked to deforestation in Indonesia, potentially avoiding 12,000 hectares/year of biodiversity loss.
SSBsโ solid electrolytes complicate recycling processes. Toyota is developing hydrometallurgical methods to recover lithium and sulfur, aiming for 90% material reuse by 2035.
LIB recycling is more established, with LFP (lithium iron phosphate) batteries achieving 70% recovery rates.
Global Economy and Tariff Implications
The U.S. Inflation Reduction Act (IRA) and EU Battery Regulation prioritize localized SSB production to avoid reliance on Chinese LIBs.
As of 2025, China produces 70% of global lithium-ion cells, but SSB patents are concentrated in Japan (Toyota, Nissan) and South Korea (LG, Samsung).
Tariffs on Chinese LIBs, such as the U.S.โs 64.5% duty, could accelerate SSB adoption by making domestic production cost-competitive.
SSB production costs are currently 2โ3ร higher than LIBs due to low yields and expensive solid electrolytes. BMW estimates SSB pack prices must fall below $80/kWh to compete with Gen6 lithium-ion batteries, which will cost $75/kWh by 2030.
Toyotaโs gigacasting strategy-using 6,000-ton presses to mold battery housings-aims to cut SSB costs by 40% through simplified assembly.
Comparative Analysis of Battery Ranges
The table below contrasts Toyotaโs hydrogen fuel cell vehicles (FCVs) with mainstream lithium-ion technologies:
Technology | Energy Density (Wh/kg) | Range (EPA) | Charging/Refueling Time |
---|---|---|---|
NMC Lithium-Ion | 250โ300 | 450โ550 km (280โ340 mi) | 20โ30 mins (DC fast) |
LFP Lithium-Ion | 150โ200 | 350โ400 km (220โ250 mi) | 30โ40 mins (DC fast) |
Toyota SSB (Prototype) | 400โ500 | 750โ1,000 km (465โ620 mi) | <10 mins |
Toyota Mirai (Hydrogen) | 1,200 (Hโ tank) | 647 km (402 mi) | 3โ5 mins |
Toyotaโs Mirai FCV exemplifies hydrogenโs rapid refueling advantage but lags in energy density compared to SSBs. The second-gen Mirai achieves 402 miles per tank, while SSB prototypes exceed 600 miles, signaling a shift toward battery dominance.
While Toyotaโs solid-state batteries offer unparalleled range and safety, scalability and cost challenges persist, with competitors like Nissan and Mercedes-Benz closing the gap. Sustainable material sourcing and geopolitical tariffs will critically influence SSB adoption, as nations vie for control over next-gen battery supply chains. While hydrogen retains niche applications, SSBs are poised to dominate the EV market by 2030, provided manufacturing breakthroughs materialize.
Article Last Updated: May 11, 2025.